by Ezio Bartocci, Oliver Hoeftberger and Radu Grosu
Cyber-Physical Systems (CPS) are the next generation of embedded ICT systems that are becoming pervasive in every aspect of our daily life. In this article we discuss some of the theoretical and practical challenges that we are currently facing in this area.
Most modern computing devices are ubiquitous embedded systems employed to monitor and control physical processes: cars, airplanes, automotive highway systems, air traffic management, etc. In the past, research on embedded systems tended to focus on the design optimization problems of these computational devices. In recent years, the focus has shifted towards the complex synergy between the computational elements and the physical environment with which they interact. The term Cyber-Physical Systems (CPS) was coined to refer to such interactions. In CPS, embedded computation and communication devices, together with sensors and actuators of the physical substratum, are federated in heterogeneous, open, systems-of-systems. Examples include smart cities, smart grids, medical devices, production lines, automotive controllers, and robotics.
Here we discuss some of the research problems that we are addressing on both the theory and practical application scenarios of CPS, such as automotive and medical systems.
Theoretical foundations and challenges
The behaviour of CPS is characterized by the nonlinear interaction between discrete (computing device) and continuous phenomena (the physical substratum). For this reason, research on hybrid systems plays a key role in modelling and analysing CPS. CPS are usually spatially distributed and they exhibit emergent behaviours (i.e. traffic jams, cyber-attacks), which result from interactions among system components, and which cease to exist when specific components are removed from the systems. Owing to their ubiquity and impact on every aspect of our life, one of the greatest challenges of this century is to efficiently predict the emergent behaviours of these systems. The complexity of their models, however, often hinders any attempt to exhaustively verify their safe behaviour.
An alternative method is to equip CPS with monitors and to predict emergent behaviours at runtime. This approach makes CPS self-aware, opening up new approaches to designing systems that can dynamically reconfigure themselves in order to adapt [1] to different circumstances. However, monitoring introduces a runtime overhead that may alter the timing-related behaviour of the system under scrutiny. In applications with real-time constraints, overhead control strategies may be necessary to reduce the overhead to acceptable levels by, for example, turning on and off the monitoring. Gaps in monitoring, however, introduce uncertainty in the monitoring results. Hence, our current research [1] also focuses on efficient techniques to quantify this uncertainty and compute an estimate of the current state of the system.
Automotive scenario
The extensive integration of sensor networks and computational power into automotive systems over recent years has enabled the development of various systems to assist the driver during monotonous driving conditions, and to protect the passenger from hazardous situations. This trend will inevitably lead to autonomous vehicles. In order to plan actions and reliably negotiate traffic, these vehicles need sensors capable of fault tolerant observation of their environment. Additionally, vehicle to vehicle (V2V) and vehicle to infrastructure (V2I) communication technology – also known as V2X communication – will be integrated into future automotive systems. V2X communication allows exchange of information between vehicles and roadside units about position and speed of vehicles, driving conditions on a particular road, accidents, or traffic jams. Information exchange thereby allows traffic load to be distributed among several roads during rush hour, as well as preventing accidents and multiple collisions and sending automated emergency calls. Figure 1 schematizes different levels of an automotive system-of-systems consisting of sensor networks within a car, and the interaction of vehicles on a higher system level.
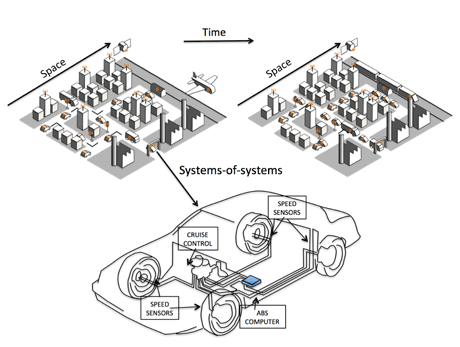
Figure 1: Multi-level CPS in the automotive domain.
Fast error detection, fault tolerant system designs and new planning strategies are required to cope with the increasing failure rates of microchips owing to continuous shrinking of devices, as well as reliance on unreliable sources of information (e.g., information sent by other vehicles). Some of these problems can be solved by knowledge-based techniques, such as autonomous reconfiguration and substitution of faulty subsystems and components by using system ontologies [2].
Medical cyber-physical systems
Medical CPS refers to modern medical technologies in which sophisticated embedded systems equipped with network communication capabilities, are responsible for monitoring and controlling the physical dynamics of patients’ bodies. Examples include proton therapy machines, electro-anatomical mapping and intervention, bio-compatible and implantable devices, and robotic prosthetics. Malfunctioning of these devices can have adverse consequences for the health of the patient. The verification, validation and certification of their reliability and safety are extremely important and still very challenging tasks, owing to the complexity of the involved interactions. The modelling and efficient simulation of the patient body will play a key role in the design and validation of Medical CPS and in the development of personalized treatment strategies. To this end, our research has largely focused on modelling and analysis techniques for cardiac dynamics to predict the onset of arterial and ventricular fibrillation. In [3] we show that with a normal desktop with GPU technology, it is possible to achieve simulation speeds in near real-time for complex spatial patterns indicative of cardiac arrhythmic disorders. Real-time simulation of organs without the need for supercomputers may soon facilitate the adoption of model-based clinical diagnostics and treatment planning.
Link: http://ti.tuwien.ac.at/cps
References:
[1] E. Bartocci et al.: “Adaptive Runtime Verification”, in proc. of RV 2012, LNCS, vol. 7687, Springer, pp. 168-182, 2012
[2] O. Höftberger, R. Obermaisser: “Ontology-based Runtime Reconfiguration of Distributed Embedded Real-Time Systems”, in proc. of ISORC 2013
[3] E. Bartocci et al.: “Toward real-time simulation of cardiac dynamics”, in proc. of CMSB '11, New York, NY, USA, pp. 103-112, 2011.
Please contact:
Radu Grosu
Vienna University of Technology
E-mail:
http://ti.tuwien.ac.at/cps/people/grosu
Ezio Bartocci
Vienna University of Technology
E-mail:
http://www.eziobartocci.com
Oliver Hoeftberger
Vienna University of Technology
E-mail: