by Antoine Chaillet, Diane Da Silva, Georgios Detorakis, Christophe Pouzat and Suhan Senova
By allowing a precise stimulation of targeted neurons through light impulses, optogenetics is revolutionizing our understanding of the brain. Its recent use in primate deep brain structures promises unprecedented data to model the mechanisms underlying Parkinson’s disease symptoms and to improve their treatment by electrical stimulation.
Neuronal synchronization plays a central role in brain functioning. It is linked to memory, cognition and movement path generation. Abnormal synchronization in certain cerebral zones can also lead to pathological states such as Parkinson’s disease, essential tremor, or epilepsy. In particular, Parkinsonian symptoms are known to be linked to persistent beta band (13-30Hz) oscillations in deep brain structures called basal ganglia.
Deep brain stimulation (DBS) is a symptomatic treatment of several synchronization-related neurological diseases [1]. It consists of electrical stimulation of deep brain structures through permanently implanted electrodes. In most existing DBS techniques, the stimulation is a square signal (1 to 5V amplitude at around 120 to 180Hz) whose precise parameters are derived primarily by trial-and-error on each patient. It operates in an open loop: no cerebral information or models of the dynamics involved are exploited.
Despite its strong success and impressive efficacy in most cases, current DBS treatment still suffers from considerable limitations, including: the spread of electrical stimulation within non-targeted brain tissues; and the low level of knowledge about the exact functioning of DBS. More importantly, a number of side effects have been reported, such as cognition issues, depression, speech disturbances, and balance impairment. Moreover, the permanent stimulation of deep brain structures leads to an overuse of embedded electrical resources, which in turn imposes further surgical operations for battery replacement. Finally, the trial-and-error selection of the present DBS parameters has been effective because of the almost immediate effects of DBS on Parkinsonian motor symptoms; new therapies utilizing DBS technology will not allow such a tuning (for instance, the beneficial effects of stimulation can take weeks to manifest in dystonia or obsessive compulsive disorders).
These limitations seem strongly linked to the disproportionate signal amplitude and the open-loop nature of the current DBS signals. Control engineering tools may contribute to a better understanding of basal ganglia circuitry and the adaptation of DBS signals to real-time cerebral activity. These are the keys for the development of more adaptive and parsimonious stimulation policies.
The recent technique of optogenetics provides unprecedented neurophysiological data, which may improve our understanding of basal ganglia interaction. It relies on a gene transfer technology to make targeted neurons sensitive to photostimulation by visible wavelengths after the selective transfer of genes coding for the expression of photosensitive ionic channels on neuronal membrane [2](Boyden et al. 2005). Thus, simple pulses of intense light, for instance through implanted optical fibers, can induce the response of the photosensitized selected neurons only, while not affecting neighbouring neurons. This technique enables an accurate spatiotemporal neuromodulation at the level of the millisecond and of one targeted neural network. Combined with electrode measurements, optogenetics offers unprecedented possibilities to dissect basal ganglia mechanisms in health and disease, and thus to optimize DBS treatment.
The SynchNeuro project, started in 2013 and co-financed by the French ANR and the Digiteo research network, proposes a multidisciplinary research effort to investigate methodologies for the analysis and control of neuronal synchronization and their application to the treatment of neurological diseases by closed-loop DBS, based on optogenetics data. The aim is to understand the underlying neurological phenomena and to develop closed-loop real-time DBS controllers that intelligently and autonomously alter pathological oscillations.
More precisely, four objectives will be addressed. First, a firing-rate model of basal ganglia under photostimulation will be developed based on in vivo primate data. This modelling and identification will be performed on both healthy and Parkinsonian primates. In order to interpret the neurophysiological data acquired by optogenetics and in vivo extracellular recordings, the raw data needs to be processed and analysed. This involves the implementation of a spike sorting method [3] and the development of a stochastic model for parameter identification. Those parameters will later be used to develop a more precise model of the neural populations, including the specificity of photostimulation. Second, based on this firing-rate model, analytical conditions for the pathological oscillations onset will be developed using tools from nonlinear control theory. Third, innovative closed-loop DBS signals that adapt in real-time to the recorded brain activity will be designed in order to attenuate the pathological oscillations. These DBS signals are expected to be more efficient and more respectful than existing open-loop DBS strategies. Finally, both the population model and the developed DBS signals will be validated numerically and in vivo. The overall setup is reported in Figure 1.
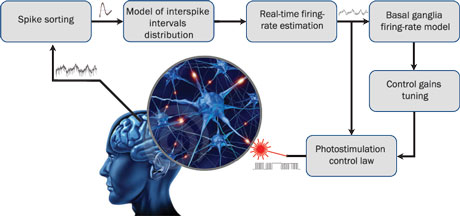
Figure 1: Schematic description of the SynchNeuro strategy. Electrical measurements of single spike recordings in specific brain structures, thanks to permanently implanted electrodes, allow to estimate the firing rate of the whole neuronal population after spike sorting and probabilistic identification. The estimated firing rate is then used off-line to identify the dynamical properties of the neuronal activity and to deduce the optimal tuning of the control gains. It is also used on-line to provide a real-time photostimulation feedback, using optogenetics, to reduce the magnitude of pathological oscillations.
By adapting control techniques and theoretical background to the specific constraints imposed by DBS (model imprecision, limited measurements and actuation, nonlinearities), decisive improvements in terms of adaptation, side effects and energy consumption can be expected. The optimization of the DBS technique is a perfect illustration of the gains that can be achieved through a closer interaction between control engineering and neuroscience.
Acknowledgement: R. Aron-Badin (CEA MIRCen) and P. Hantraye (CEA MIRCen), L. Greco (L2S) and S. Palfi (APHP H. Mondor hospital) are also partners of the SynchNeuro project and have contributed to this article. This work has received support from the French ANR project SynchNeuro, the French Digiteo project NEUROSYNCH, the French institute iCODE, and the European FP7 Network of Excellence HYCON2.
References:
[1] A.L. Benabid et al.: “Long-term suppression of tremor by chronic stimulation of the ventral intermediate thalamic nucleus”, The Lancet, 337(8738), pp.403–406, 1991
[2] E.S. Boyden et al.: “Millisecond-timescale, genetically targeted optical control of neural activity”, Nature Neuroscience, 8(9), pp.1263–1268, 2005.
[3] C. Pouzat, O. Mazor, G. Laurent: “Using noise signature to optimize spike-sorting and to assess neuronal classification quality”, journal of neuroscience methods, 122, pp.43–57, 2005.
Please contact:
Antoine Chaillet
L2S, University Paris Sud 11,
Supélec, France
E-mail: