by Miguel Jiménez Carrizosa, Yijing Chen, Gilney Damm, Abdelkrim Benchaib and Françoise Lamnabhi-Lagarrigue
The control of Multi-Terminal High Voltage Direct Current (MT-HVDC) networks is still an open problem. Communication within this wide-span network is a very delicate matter: the time delay and possible loss of information must be taken into account. We are aiming to develop and control a hierarchical stable structure that manages such a network.
Power systems’ networks are among the largest and most complex physical man-made systems. They connect hundreds of millions of producers and consumers, cover continents and exhibit very complicated behaviours. Many poorly-understood phenomena result from the interactions between such a large number of devices and the large spatial dimension, and as a consequence their management and stabilization may be very challenging. At the same time, the dependence of modern societies on electricity is growing, with our reliance on pervasive computers, embedded systems and cellular devices. This dependence will most likely continue to grow if a lot of electric vehicles become a reality. For these reasons the impact of disturbances and blackouts has become astronomic.
Major changes are putting the power grid under great stress; in particular the large scale introduction of renewable energy sources is a major cause. The goal of reducing carbon dioxide emissions and creating a greener world will lead to an increased use of renewable energy sources (RES) in the form of wind farms or solar plants. Two distinctive features of these energy sources present an important challenge: most RES are dispersed over a very wide geographical area, and most RES are intermittent. Since further development of the classical Alternative Current (AC) Grid will not be possible owing to economic (in the offshore case) and environmental issues, the only foreseeable solution becomes the use of Direct Current (DC) links, and most probably a new DC off-shore Grid interconnecting AC grids or other DC grids [1], constructing a new SuperGrid. Integrating these new technologies into the existing AC grid and introducing new tools for handling specific control problems related to the distributed control of large offshore wind farms interconnected by a DC network, as well as the connection of this DC network to the AC grid is currently under investigation.
Recently, multi-terminal HVDC (MT-HVDC) networks which consist of more than two converter stations have drawn more and more attentions. The MT-HVDC network offers a larger transmission capacity than the AC network and provides a more flexible, efficient transmission method. The main applications of MT-HVDC networks include power exchange among multi-points, connection between asynchronous networks, and integration of scattered power plants like offshore renewable energy sources. The control of a Multi-Terminal High Voltage Direct Current (MT-HVDC) network, as shown in Figure 1, is still an open problem. We are developing a hierarchical structure to manage such a network. The wide-span makes communication a very delicate matter. The time delay and possible loss of information must be taken into account when managing the network. It is therefore necessary to rely on lower level local only controllers [2], which will stabilize the system against changes and disturbances in the network.
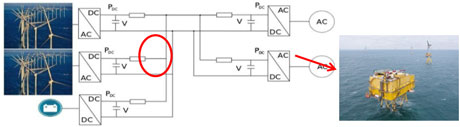
Figure 1: An MT-HVDC system (left) and an offshore converter station.
Local controller
The lower level focuses on designing a local controller on each node in an MT-HVDC system, without using remote information. Its first objective is to make the converter accurately track its reference values which are provided by a higher level controller. In addition, in case of disturbances such as power imbalance, the system must be always kept stable and quickly converge to a new steady state condition. Finally, in a second step, the local controller should be driven by the higher level controller, which maintains sustainable operating conditions.
Most current research is based on Voltage Source Converters (VSC), and in particular with MMC (Modular Multilevel Converters) technology. The behaviour of a VSC terminal can usually be characterized by its DC and AC subsystems, which constitute its state vector (in particular the AC currents and DC voltage). Numerous control strategies for VSC-HVDC systems have been developed recently. Most, however (for example [3]), are based on intuitive assumptions without rigorous mathematical demonstrations.
Our group is investigating several control approaches to improve system performance via different control theories and rigorous model-based analysis.
There are three on-going approaches:
- Nonlinear control based on feedback linearization: although some linear system properties can be applied to regulate the behaviours of the system, the main drawback is that the system can only operate in unidirectional mode.
- Nonlinear control based on static and dynamic feedback linearization: this novel controller has been developed based on a static and on a dynamic feedback linearization structure in order to operate in bidirectional mode. For example, when the terminal absorbs the power from the DC grid it uses a static part; otherwise it uses the dynamic part. However, this method involves a switch system according to the power direction, which could present undesirable behaviours such as chattering and the appearance of large peaking values.
- Globally stable passive control [1]: the main advantage of this controller is that global stability is guaranteed. In addition, the VSC terminal can operate more smoothly than the other approaches.
Primary (Droop) controller
Droop control reacts to a power imbalance, and can be explained as follows. When there is a power variation in one or several nodes, the other nodes will adjust the amount of generated/consumed power via droop control, by adjusting their voltages, see Figures 2(a) and 2(b). This control is implemented as a set of local proportional controllers with gains called the droop gain. These local gains are represented by the slope shown in Figures 2(a) and 2(b). This value, which depends on several variables, such as the size of each node and its available (primary) energy reserve, indicates how much power each generator provides when a variation occurs, in function of its capabilities.
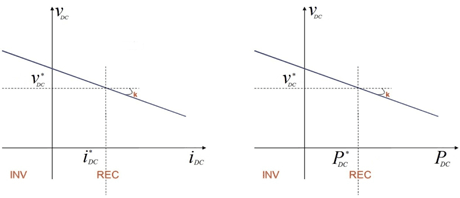
Figure 2(a) (left) and 2(b): Droop technique for primary control strategy.
Secondary controller
The main objectives of the secondary control are: to follow the tertiary level references; and to make a periodic power flow in order to guarantee the proper behavior of the system. The secondary control is also responsible for predicting and avoiding congestion in the lines.
When a disturbance occurs in the grid, it is the job of the secondary control to restore the equilibrium, taking into consideration the current energy reserves. To achieve this, communication between nodes is necessary. In this respect, the secondary control differs from the primary control, in which no communication occurs. The secondary control is a critical element in the proper functioning of the system because it takes into consideration disturbances as well as load forecast. It has information on the current and future short-term state of the system, and it creates the link between the physical system, in the timeframe of milliseconds, and the economic forecast that is in the order of hours. Its role is then to calculate voltage values to optimize the power flow to minimize transmission losses, for example, subject to all the constraints. These voltage values are then transmitted as references for the primary level.
To develop the secondary controller we have used Model Predictive Control (MPC), because it can provide an optimal solution taking into account these constraints whilst also considering forecasts, such as weather, load, or even prices.
In summary, the lower level controllers stabilize the system in the face of changes within the system as well as large disturbances, while higher level intercommunicating controllers steer the overall system to reliable and optimal operating conditions.
Link: http://www.eeci-institute.eu/WINPOWER
References:
[1] M. Jiménez Carrizosa, et al.: “Bilinear and nonlinear control algorithms for a DC/DC converter for multi-terminal HVDC networks”, IFAC World Congress 2014
[2] Y. Chen, et al.: “A globally stable passive controller for a VSC terminal in a multi-terminal HVDC grid”, in proc. of ACC’14, Portland, USA
[3] J. L. Thomas , S. Poullain, A. Benchaib: “Analysis of a robust DC-bus voltage control system for a VSC transmission scheme”, in proc. of the 7th Int. Conf. AC-DC Power Transmiss., 2001.
Please contact:
Gilney Damm
Laboratory IBISC, France
E-mail:
Abdelkrim Benchaib
ALSTOM GRID, FRANCE
E-mail:
Françoise Lamnabhi-Lagarrigue
LSS-SUPELEC, France
E-mail: