by Stefano Savazzi, Vittorio Rampa and Umberto Spagnolini
Strong fluctuations in crude oil prices and the expected production peak of current reservoirs are pushing oil companies to increase their investment in seismic exploration. Replacing cabling with wireless technology should radically improve the quality of depth imaging and simplify acquisition logistics. Recent advances in Wireless Sensor Networks (WSN) now allow the wireless community to satisfy the rigid constraints imposed by seismic acquisition systems, which have a large number of sensors (> 10 000) over the monitoring area (> 5km2).
Strong fluctuations in crude oil prices are pushing oil companies invest more in seismic exploration of new oil reservoirs and in new technology to improve the quality of depth imaging. Seismic prospecting requires a large number of sensors (up to 30 000), such as geophones or MEMS-based (Micro Electro-Mechanical Systems) accelerometers. These are deployed over large areas (up to 30km2) to measure the back-scattered wavefield generated by an active excitation source. A storage/processing unit (sink node) collects measurements from all the geophones in real time to obtain an image of the sub-surface. Current telemetry is cable-based and usually requires hundreds of kilometers of cabling, which results in delays, high logistic costs and low imaging quality.
Wireless technology is thus expected to significantly improve the efficiency of oil exploration. Technical limitations in the data-rate efficiency, interference and battery use of current short-range wireless network architectures (eg WiFi, Bluetooth) forced previous proposals for wireless geophone system architectures to choose a combination of wireless and wired configuration. However, recent advances in WSN technology conveniently address the issues related to the strong constraints imposed by seismic acquisition systems. A Wireless Geophone Network (WGN) must support multiple acquisition settings and applications. Basic network requirements are: i) network throughput of 150kbps down to 50kbps for single component sensors; ii) real-time (or near real-time) acquisitions with strong delay constraints; iii) remote control by sink node and synchronous acquisition with a maximum timing skew of 10ms; and iv) accurate positioning of each sensor/geophone with an error of less than 1m to avoid degradation of the depth imaging quality.
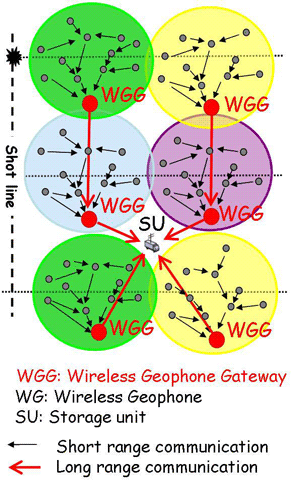
Network Architecture
As shown in Figure 1, the proposed WGN architecture exploits different radio transmission technologies to efficiently handle both short-range transmissions (ie for short-distance low-power communication among geophones/sensors), and long-range transmissions (ie for seismic data delivery to storage units and geophone remote monitoring) that must cover distances of several kilometers. The hierarchical network design requires the deployment of a number of Wireless Geophone Gateways (WGGs) to collect data readings from a large number of wireless geophones (WGs) and forward the data to the storage unit (SU). These WG nodes are self-organized into independent sub-networks; ideally the number of devices per sub-network should be as high as 300 nodes to minimize the number of WGGs. This results in an aggregated (per sub-network) throughput of about 45Mbps (up to 60Mbps). Data delivery within one sub-network is obtained by multi-hop transmissions towards the WGGs; WG sensors are within 5-100m of inter-node distance to reduce both energy consumption and increase battery life.
Physical and MAC Layer Requirements
The requirements of self-localization and frame synchronization make Ultra WideBand (UWB) technology the natural choice for short-range transmissions within each sub-network. To achieve positional accuracy with errors less than 1m, the travel-time estimation error for ToA-based (Time of Arrival) positioning must be in the order of 3ns with a minimum required signal bandwidth of 500MHz. UWB technology provides data acquisition, synchronization and localization without the use of fully GPS-based (Global Positioning System) WGN nodes. Moreover, recent advances in radio design (ie MB-OFDM or MultiBand Orthogonal Frequency Division Multiplexing) provide wireless devices with high data rates over short ranges of up to 480Mbps, and low power consumption (ie below 100mW in active transmission mode but down to 20mW in power-save mode). The MB-OFDM processing can also guarantee network scalability through time and frequency division by allowing the use of multiple sub-bands to separate the co-located sub-networks, and coexistence with other 2.4 GHz-based radio devices without significant cross-interference.
The high number of devices per sub-network and the large network size suggest the adoption of a number of distributed MAC (Medium Access Control) functionalities. Network topology should define a hierarchical structure where the WGG acts as an intermediate sink towards the storage unit. The WiMedia standard (ECMA-368 from ECMA International, the European Association for Standardizing Information and Communication Systems) has been chosen as the reference for the development of the WGN MAC. Transmission is organized in superframes with the beacon period (BP) carrying the essential information of each device. Logical device/sensor groups are dynamically formed according to WiMedia protocol to facilitate the sharing of resources, while wireless medium reuse can be exploited over different spatial regions.
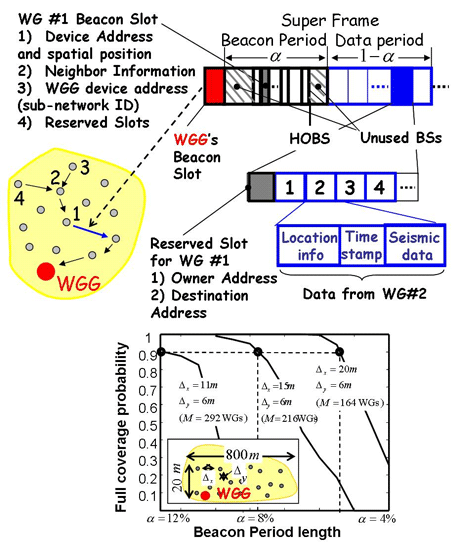
WGG supports specific extended functions compared to a standard WiMedia device. These functions allow: i) the Gateway to behave as an intermediate sink, forwarding data to the storage/ processing node SU and controlling each sub-network; ii) contention-free resource negotiations to guarantee real-time constraints (eg quality of service and maximum delay); and iii) coexistence of long/short range transmissions. Figure 2 illustrates the MAC layer framing structure adopted for each sub-network, while the probability of full network coverage versus the BP length is shown at the bottom of the same figure. Sensors/geophones are assumed to be deployed according to the requirements of a conventional seismic survey. Geophone deployment has a major impact on the framing structure design: a lower density with a sensor spacing of 20m (still reasonable for seismic acquisitions) can make WiMedia feasible for WGN applications with minimal modifications. On the other hand, higher geophone densities, with a sensor spacing of less than 11m, require further MAC modifications. More details are given at the WisyGeo Web site.
Links:
Land seismic exploration: http://www.oilfieldreview.com/
Wireless Geophone Network:
http://www.wisygeo.com
http://scitation.aip.org/tle
WiMedia: http://www.wimedia.org
Please contact:
Stefano Savazzi, Umberto Spagnolini,
DEI, Politecnico di Milano, Italy
E-mail: savazzielet.polimi.it, uspagnolini
elet.polimi.it
Vittorio Rampa
IEIIT-CNR, Italy
E-mail: rampaelet.polimi.it