In recent years there has been a rapid growth in the understanding of the basic cellular processes of individual bacterial cells through advances in genomics and proteomics research. However, this has introduced a demand to understand how the interactions between the individual system components contribute to the overall population dynamics, which is of great relevance in both ecological and clinical studies. A useful theoretical approach for relating information at the individual cellular/molecular level with emergent population characteristics is the agent-based (or individual-based) modelling approach.
The agent-based modelling approach involves assigning pre-defined rules and parameters to each individual component (eg the bacterial cell) of the population. Therefore, the emergent behaviour of the population as a whole can be examined without the need for population-level laws. This allows the inherent heterogeneity of a population to be accounted for in the model, by explicitly modelling local differences in the environment and between different bacterial cells. This approach differs from the traditional 'top-down' mathematical modelling approaches which represent the growth of the colony as a whole as a function of the normal rate of growth of the bacteria assuming population-averaged parameters.
An agent-based model of bacterial population growth, called the Micro-Gen Bacterial Simulator, has been developed at the Centre for Scientific Computing & Complex Systems Modelling (SCI-SYM) in Dublin City University, Ireland. Its aim is to provide a theoretical framework for investigating the growth and development of bacterial colonies and their interactions with antibiotics. The project has been carried out in close collaboration with Dr. Marc Devocelle at the Centre for Synthesis and Chemical Biology in the Royal College of Surgeons in Ireland (RCSI), Dublin, and his collaborators from the Clinical Microbiology laboratory at Beaumont hospital, Dublin, Ireland.
The simulated culture environment of Micro-Gen is represented by a discrete, two-dimensional grid containing diffusible elements such as nutrients, enzymes and antibiotics that the bacterial cells can interact with. The individual bacteria of a colony are represented by software agents, which store the physical traits such as energy state or antibiotic damage and the behavioural rules of the bacteria. The model is adaptable to represent a wide variety of different bacterial species. Figure 1 shows a screenshot from Micro-Gen of bacterial colonies (yellow circles) growing on nutrient agar medium (blue, lighter shade represents higher nutrient concentration).
Micro-Gen is designed to take advantage of parallel computing resources by dividing the simulation environment equally among individual nodes in a multi-processor cluster. The model is highly scalable in order to be able to represent the large concentrations of bacterial cells typically found in natural colonies (>107 bacteria per millilitre). This is an important factor to consider because the number of interacting cells in a bacterial colony has been shown to have a significant impact on the colony behaviour, especially in its response to antibiotic treatment (so-called 'inoculum effect').
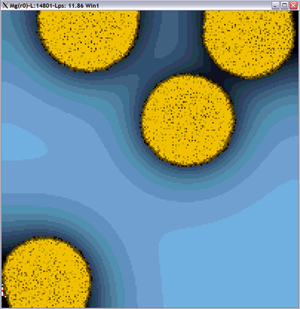
A key component of the model is the ability to quantitatively model antibiotic molecules and their interactions with the bacterial cells. These interactions are governed by defined kinetic parameters specific to the type of antibiotic and bacterial strain being modelled. This allows a quantitative model of antibiotic interactions with bacteria to be built up and their pharmacokinetic properties to be investigated.
The model also incorporates two important antibiotic resistance mechanisms employed by bacterial cells: (A) Special enzymes released by bacteria, called
b-lactamases, which degrade the antibiotic molecules; (B) Reduced binding affinities between the antibiotics and receptors in the bacterial cells. These antibiotic resistance mechanisms are of great clinical concern as their development and spread across many species of bacteria has led to the erosion of the efficacy of many commonly prescribed antibiotics, in particular penicillin and its derivatives.
Tests were carried out to validate the Micro-Gen model by using kinetic parameters applicable to some common antibiotics (penicillin, ampicillin, and cephalothin) versus the clinically important multi-drug resistant bacterial strain methicillin-resistant Staphylococcus aureus (MRSA). The cellular and molecular parameters for this model were derived from the biological literature for three different strains of MRSA, and the model was used to predict the Minimum Inhibitory Concentration (MIC), which is a key clinical measure of antibiotic efficacy, of each antibiotic versus the three strains. The MIC of an antibiotic is the minimum effective dose of antibiotic that will succeed in inhibiting the growth of the bacterial colony. When the predicted MICs from the model were compared with experimentally derived MICs for MRSA, they were found to be in close quantitative agreement.
As such, the model represents a good tool for informing antibiotic treatment strategies since it can be used to investigate the principal parameters affecting antibiotic resistance in bacteria and relate this to key clinical indicators such as the MIC of a drug. There is a significant logistical burden associated with growing bacterial cultures and testing novel candidate drug compounds in the lab. However, the ability to simulate a wide variety of different conditions and parameters in a simulated environment could be used to inform rational drug design strategies.
In conclusion, Micro-Gen represents a robust tool for modelling the complex interactions of bacterial cells in colonies and their interactions with extra-cellular molecules such as antibiotics. In particular, the ability to investigate the antibiotic efficacy and predict an important clinical parameter such as the MIC using basic low-level cellular/molecular information represents a significant, novel contribution to the field.
Future work will include expanding the model to represent more complex, three-dimensional bacterial communities, such as biofilms, that form in nature. In these structured communities, dynamic community interactions take place between multiple species of bacteria forming a complex interdependent microbial ecosystem. The agent-based modelling approach is particularly suited for modelling highly heterogeneous and dynamic structures such as these.
Links:
http://www.computing.dcu.ie/~jamurphy/
http://sci-sym.computing.dcu.ie/
Please contact:
James T. Murphy
Dublin City University, Ireland
Tel: +353 1 700 8449
E-mail: jamurphycomputing.dcu.ie